As world leaders in plant genome research, Cornell University, Boyce Thompson Institute (BTI), the USDA-ARS, and the U.S. Plant, Soil, and Nutrition Laboratory are host to many outstanding research labs. These research facilities have built on Cornell’s long tradition of research in plant genetics and breeding to develop novel technologies, the application of which has sought to improve the scientific understanding of many aspects of plant biology. The research interests of the labs are quite varied, ranging from identifying disease resistance in crop plants to understanding how plants sense and respond to light. Please click the following text to learn more about the faculty members associated with the research projects in the various summer internship opportunities at BTI.
Internship Projects
To learn more about available projects and their faculty sponsors, click on the topics below.
Chemical ecology and coevolution of monarchs and milkweeds - Agrawal Lab, Cornell
Project description:
Our lab studies the ecology and evolution of plant-insect interactions, including aspects of plant defense (the spines and toxins plants produce to reduce herbivory), induced defense (the immune-like system of plants in response to attack), chemical ecology (how interactions between species are mediated by chemistry), and coevolution (long-term reciprocal adaptation and “arms race” evolution when species battle). We work on local biodiversity and especially milkweed plants and monarch butterflies.
Approaches in our lab are diverse, and typically involve field research, chemical analyses, genetic techniques, and rearing lots of bugs. Our work has advanced both basic questions in ecology, evolution, and plant biology, as well as applications to insect pest management (especially in cucurbit crops) as well as conservation biology (of monarch butterflies).
For more information about Agrawal’s lab, publications, and his blog please click here
Faculty advisor: Anurag Agrawal
Molecular Definition of Plant Organelle Editosomes - Bentolila Lab, Cornell
Project description:
Among post-transcriptional processes that affect gene expression, C-to-U RNA editing in plants exhibits a number of unique features. Editing is confined to the genome-containing plant organelles—chloroplasts and mitochondria. Unlike other types of RNA editing, the purpose of plant RNA editing is not to create multiple proteins from the same transcript—instead, its role is to correct T-to-C mutations in critical locations of transcripts in order to produce functional proteins. A typical vascular plant requires over 600 RNA editing-mediated corrections in its organelle transcripts. A combinatorial approach involving quantitative genetics, biochemistry and genomics has allowed us to identify several components of the editosome, the editing machinery responsible for the modification of C to U on organelle transcripts. In addition to PPR proteins, which act as recognition factors controlling the specificity of the editing reaction, we have identified three other protein families as providing components to the plant editosome. Ultimately the goal of this research is to reconstitute the plant editosome in vitro, and from there pave the way for future genetic engineering of RNAs in plants and other organisms, technology which could benefit the biotechnology industry and society.
Faculty advisor: Stephane Bentolila
TAL effector biology for durable plant disease prevention and DNA targeting applications - Bogdanove Lab, Cornell
Project description:
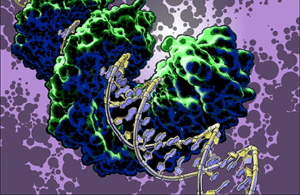
TAL effector PthXo1 bound to its target. Illustration by Jon Bogdanove
Research in the Bogdanove laboratory is directed at understanding mechanisms of bacterial plant pathogenesis and plant defense to develop better means of disease control. We focus in the interactions of plant pathogenic bacteria in the genus Xanthomonas with important crop hosts. TAL effectors are injected into plant cells by the bacterium, enter the nucleus, and activate specific plant “susceptibility” (S) genes that contribute to disease development. TAL effectors recognize their DNA targets in a modular way: tandem, variable structural repeats in these proteins independently specify single contiguous bases in the DNA. This correspondence makes it possible to rapidly identify TAL effector targets, to engineer novel TAL effectors with custom assortments of repeats to bind DNA sequences of choice, and even to customize genes for TAL effector activation (or prevent it!). Major areas of activity in our lab include 1) identification and characterization of Sgenes, 2) genomic analyses to identify the diversity of TAL effectors in pathogen populations and understand their evolution, and 3) structural and biochemical studies to better harness the unique properties of these proteins for applications such as targeted gene regulation and genome editing. REU opportunities in the lab are available in each of these areas.
Faculty advisor: Adam Bogdanove
Virus recognition of insect vectors and collaborations - Casteel Lab, Cornell
Project description:
Numerous studies demonstrate that vector-borne pathogens, such as viruses, influence host characteristics that result in altered host-vector interactions and enhanced virus transmission. We recently demonstrated that viral proteins respond actively to the presence of insect vectors, promoting insect performance and transmission only when needed. We seek to determine the molecular mechanisms that underlie this phenomenon and use this knowledge to develop innovative control strategies using genetic and biochemical approaches. Current focuses are on changes in plant protein turnover and defenses, cell biology, chemical ecology, and protein functions in response to virus infection and insect vectors. Students will receive training in molecular biology, chemistry, genetic engineering, and ecology.
Faculty advisor: Clare Casteel
Investigating the molecular mechanisms underlying fruit set and development- Catala Lab, BTI
Project description:
Fruit development is a crucial process in the sexual reproduction of flowering plants and of critical importance for seed dispersal, plant fitness and agricultural yield. Fruit are complex organs that arise from the coordinated growth and development of floral tissues following pollination. Research in the Catala lab focuses on the molecular regulation of fruit formation and early development using tomato as a model system. We use molecular and genetic techniques to investigate the complex interplay of gene expression changes, signaling events, and hormonal activity, controlling fruit development. The lab also studies the effect of drought stress, an increasing problem in crop production, on tomato fruit set and growth. We are taking advantage of the genetic diversity of wild tomato species, to examine the molecular basis of adaptations to water stress as well as of other fruit quality traits.
Students participate in projects aimed to identify new genes, small molecules or chemical signals playing a key role during fruit initiation. One of these projects involves the functional characterization of an ovule specific small secreted protein (OSP), specifically expressed in the inner ovule integument of tomato ovaries. OSP belongs to the cysteine-rich peptide class of small, secreted peptides, which have been involved in short-term signaling. We hypothesize that OSP, produced in the tomato female gametophyte, may participate in signaling events regulating pollen tube guidance, sperm reception and gamete activation, or in embryo development after fertilization. To characterize the function of OSP, the students involved in this project will use a range of techniques such as gene expression analysis by quantitative PCR, CRISPR-mediated gene editing, and protein localization using confocal microscopy.
Faculty advisor: Carmen Catalá
Bioinformatics and genomics to understand important traits of agricultural crops - Fei Lab, BTI
Using integrated bioinformatics and genomics approaches to understand important traits of agricultural crops.
Project description:
The development of high throughput technologies has given rise to a wealth of information at system level including genome, transcriptome, proteome and metabolome. However, it remains a major challenge to digest the massive amounts of information and use it in an intelligent and comprehensive manner. To address this question, Dr. Fei’s group has focused on developing computational tools and resources to analyze and integrate large scale “omics” datasets”, which help researchers to understand how genes work together to comprise functioning cells and organisms.
Faculty advisor: Zhangjun Fei
Quantifying the influence of flower color on plant-pollinator interactions in soybean - Frank Lab, Cornell
Project description:
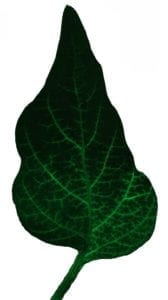
Xylem-mobile dye moving through a pepper leaf (image credit: Hannah Thomas)
The production of hybrid seeds has been used for over a century to increase crop yields, improve abiotic and biotic stress tolerance, and enhance nutritional quality. Soybean, one of the most important crops in the US, is a self-fertilizing plant with untapped yield and heterotic trait potential. Using a biotechnology approach, the Frank lab is working to transform soybean into an outcrossing crop that can benefit from hybrid breeding. One essential component of this work involves attracting bee pollinators that can promote outcrossing. In this project, students will use plant genetics, video imaging, computational modeling, and microscopy to test the influence of altered floral traits in bee visitation in greenhouse and field settings.
Faculty advisor: Margaret Frank
Illuminating belowground environments by harnessing plant metabolites for programmable plant phenotyping - Margaret Frank (Plant Biology) and Sijin Li (Chemical Engineering)
Project Description
Soil is composed of a complex matrix of organic and inorganic matter. This heterogeneous landscape can have a tremendous impact on plant performance, and yet it remains difficult to quantify the multi-partite, complex interactions between plants and their terrestrial environment (e.g., nutrients, microbes, pests, and neighboring plants). Here, we are developing a transformative strategy that combines a synthetic biosensor that can detect root-derived chemicals that are synthesized in response to specific environmental stresses and a root-to-shoot signaling system that interacts with our biosensor to transmit belowground in planta responses into the aerial half of the plant, in real-time. This approach will make belowground root phenotypes accessible to high throughput, non-destructive aerial phenotyping approaches. Two students who will be jointly supervised by Dr. Sijin Li and Dr. Margaret Frank will work closely with senior personnel on the project and will engage in collaborative research meetings between plant biologists and bioengineers. The specific tasks will involve the development and characterization of genetic biosensors and the application of plant biotechnology to harness a native root-to-shoot signaling system for above ground phenotyping. The student working on these experiments will learn about state-of-the-art synthetic biology in the Li lab, as well as biotechnology and signaling in the Frank lab.
Faculty advisors:
Carbon-concentrating mechanism in hornworts- Laura Gunn (Plant Biology) and Fay-Wei Li (BTI)
Project Description
Nature’s carbon-fixing enzyme, Rubisco, represents the major point of carbon entry into the biosphere. However, Rubisco is notoriously inefficient, exhibiting a slow catalytic rate and poor discrimination between CO2 and O2. O2-fixation leads to photorespiration, which consumes energy and releases fixed CO2. To enable more efficient photosynthesis, certain organisms have evolved biophysical carbon-concentrating mechanisms (CCM) that partition Rubisco and CO2 into a dedicated subcellular compartment. Pyrenoids are an example of such compartments – organelles comprised of an interconnected matrix of aggregated Rubisco (and associated proteins) that are liquid-liquid phase separated from the stroma (and molecular oxygen). The green alga Chlamydomonas reinhardtii has been the traditional model to study pyrenoid-based CCMs with the hope of installing a similar mechanism into crops to enhance yield. However, hornworts, a lineage of bryophytes, are the only land plants that have a pyrenoid-based CCM. Owing to their much closer relationship to crop plants, lessons learned from hornworts might have higher translational potential than those from Chlamydomonas. We are working to identify and validate putative hornwort pyrenoid components, and determine how they interact with each other in order to develop the blueprints for building a hornwort pyrenoid in crop chloroplasts.
Faculty advisors:
Laura Gunn
Fay-Wei Li
Biogenesis pathway understanding to reimagine CO2 fixation in land plant chloroplasts - Laura Gunn, Cornell
Project Description
Nature’s carbon fixing enzyme, Rubisco, is notoriously inefficient, exhibiting a slow catalytic rate and poor discrimination between CO2 and O2. O2-fixation leads to photorespiration, which consumes energy and releases fixed C O2. Accordingly, Rubisco catalysis often limits the growth rate of photosynthetic organisms, including crop species. We aim to reimagine CO2 fixation in land plant chloroplasts, however, this requires a nuanced understanding of the biogenesis pathway: we have to fully understand the pathway before we can hack it! Rubiscos from higher plants are comprised of eight catalytic large- (LSu, rbcL gene) and eight auxiliary small- (SSu, rbcS gene) subunits, which form a L8S8 hexadecamer. The nucleus of higher plants encodes an rbcS multigene family that may provide the opportunity for differential SSu expression in response to a range of intrinsic and extrinsic cues. All higher plants have one LSu-encoding gene located in the plastome and a variable number (depending on species) of nuclear-encoded SSu isoforms. We are performing experiments in planta and also by using a SynBio expression system to (i) clarify the structure-functional influence of differentially expressed Arabidopsis SSus, and (ii) investigate the mechanism underlying the incorporation of these different SSu isoforms during Rubisco biogenesis.
Faculty advisors:
Laura Gunn
Molecular and genetic analysis of fruit ripening and related nutrient pathways, using tomato as the model system - Giovannoni Lab, BTI
Project description:
Ripening is a process by which the texture, color, flavor, and nutritional content of fruit is enhanced. These traits contribute to the healthfulness and desirability of the fruit as a food source. Clearly, understanding the processes behind fruit ripening are important in terms of nutrition, but also for commercial applications such as transportation and shelf-life. Thus, the focus of research in the Giovannoni lab is molecular and genetic analysis of fruit ripening, related signal transduction systems and pathways leading to accumulation of nutritional compounds, using tomato as a model system. Researchers in the lab have characterized numerous genes related to ripening control and manifestation. In addition to identifying important genomic and regulatory components of ripening, the lab also investigates regulation of lycopene synthesis and accumulation in fruit. Lycopene is the pigment that gives tomatoes their red coloring and which is also suggested to inhibit degenerative diseases such as cancer and heart disease. Using a genomics approach, the lab is investigating the regulatory mechanisms behind accumulation of this important compound.
For more information about the Giovannoni lab, please visit the Plant Biology website. Additionally, the Giovannoni lab, in conjunction with other labs on campus has developed a resource for tomato genomics, the Tomato EST Database. Additional resources and information resulting from tomato genomics activities on the Cornell campus can be found at the Solanaceae Genomics Network site.
Faculty advisor: Jim Giovannoni
Development and testing of a worm-like, soil-swimming robot to measure plant root features and soil properties - Mike Gore (Plant Breeding) and Rob Shepherd (Mechanical and Aerospace Engineering)
Biological nitrification inhibition: Plant breeding to reduce nitrogen emissions - Gore Lab, Cornell
Project Description:
Modern agriculture’s dependence on nitrogen input has led to high yields but at the cost of eutrophication of our waterways through nitrate runoff and increased greenhouse gases through nitrous oxide emissions. A potential avenue to combat the environmental impact of nitrogen while improving yields without additional inputs is a process called biological nitrification inhibition or BNI. BNI has been established to lower nitrogen loss through specific plant root exudates that control soil microbial diversity. Yet efforts to breed BNI in crops such as maize, rice, or sorghum are slow, mainly due to the difficulties in assessing BNI capabilities. This research project combines engineering, biology, and data science to link above-ground plant traits to below-ground processes and enhance the pace at which we can select high BNI lines. The current objectives of this project are to assess the efficacy of hyper and multispectral for the prediction of BNI activity in maize and to understand how BNI activity changes throughout time.
Faculty Advisor: Michael Gore
Molecular analyses of arbuscular mycorrhizal (AM) symbiosis - Harrison Lab, BTI
Project description:
Phosphorus is a critical macronutrient for proper plant growth. While phosphorus deficiencies can be improved by the application of phosphate fertilizers, it is costly, both to the farmer and to the environment. Furthermore, the crops only take up a small percentage of the applied fertilizer; the remainder is either immobilized in the soil, or carried into ground water and rivers, often resulting in pollution.
Interns in the Harrison lab investigate two aspects of plant phosphorus nutrition. The first aspect seeks to understand the basis for the symbiotic relationships between vascular flowering plants and arbuscular mycorrhizal (AM) fungi. The fungi colonize root cells, gaining access to carbon supplied by the plant, while at the same time mobilizing mineral nutrients from the soil, including phosphorus, to be used by the plant. For this work, the lab uses the model legume, Medicago truncatula and the fungus Glomus versiforme. The Harrison lab also studies how plants find and take up phosphorus from the soil when they do not have these symbiotic relationships with fungi. This work toward understanding the mechanisms of perception and acquisition of phosphorus by plants may eventually lead to a more effective usage of fertilizers.
Faculty advisor: Maria Harrison
Understanding how insects transmit plant pathogens - Heck Lab, USDA
Project description:
The Heck lab deciphers molecular mechanisms regulating insect transmission of plant pathogens and uses this knowledge to create practical solutions that mitigate vector-borne diseases in agriculture. We use a combination of computational and wet-lab approaches to study vector-pathogen-plant interactions. Students will receive training at the intersections of computational biology, plant pathology, entomology, microbiology, genetic engineering and synthetic biology. Projects involve research on citrus greening disease or diseases caused by aphid-borne poleroviruses, such as the invasive cotton leafroll dwarf virus
Faculty advisor: Michelle Heck
Molecular genetic studies of temperature responses and immune responses in plants - Hua Lab, Cornell
Project description:
Proper responses to environmental signals are essential for plant growth, reproduction, and fitness. Understanding the molecular genetic basis of such responses is not only fundamental to the central biological question of signaling and adaptation, but also better prepares us for global climate changes.
Research programs in Hua lab include molecular genetic studies of 1) temperature regulation of plant growth, 2) regulation of plant immunity, and 3) interplay between temperature and immunity. Both induced mutations and natural variations of Arabidopsis and rice are used to dissect signaling pathways and reveal adaptive changes in signaling. These studies aim at a deeper understanding of how plants adapt and evolve in a changing environment.
Faculty advisor: Jian Hua
Genetic and biochemical mechanisms of plant defense against insects - Jander Lab, BTI
Project description:
Plants in nature are subject to attack by wide variety of caterpillars, beetles, aphids, and other insect herbivores. Although there are a million or more species of herbivorous insects, any individual plant species is resistant to the vast majority of these. Insect feeding is inhibited by an array of chemical defenses that exhibits great variability both within and among different plant species. However, although it is known that any plant leaf contains several thousand different metabolites, most of these remain unidentified. In the Jander lab we are investigating natural variation in the herbivore resistance of maize, tomato, and potato to elucidate the molecular basis of plant defense traits. Through a combination of genetic crosses, gene expression assays, metabolite profiling, and insect growth experiments, we are able to identify specific plant genes, biosynthetic pathways, and metabolites that are required to mount an effective anti-herbivore defense.
Faculty advisor: Georg Jander
Understanding stress-induced changes in plant architecture for improved resilience - Julkowska Lab, BTI
Project description:
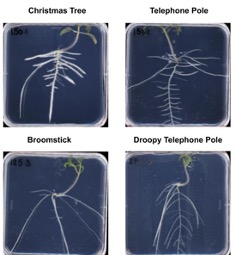
The root system architecture types that Julkowska lab has identified in wild tomato relative, S. pimpinellifollium.
Plants adjust their development to the environmental conditions. This incredible flexibility is exhibited in tropic responses, like phototropism, but also extends beyond the individual organ scale. In Jukowska lab we are interested in how environmental stress shapes plant architecture. Stress exposure often induces quiescence of growth through modification of cell cycle activity, cell expansion and cell wall extensibility. The period of initial growth arrest and the extent of recovered growth differs between individual organs leading to altered plant architecture.
Interns in Julkowska lab will explore changes to plant architecture using timeseries experiments, where the changes in plant growth are recorded using Raspberry Pi computers connected to the cameras. The images are subsequently analyzed using PlantCV software and the dynamics are examined using pipelines in R. The environmental changes are explored in domesticated plant species, such as tomato or common beans, but also across resilient species, such as Solanum pimpinellifolium, which is a close relative to cultivated tomato, but exhibits tremendous resilience to heat and drought stress, as well as cowpea, which is known for its resilience to heat and drought, and performs well in subsistence farming across the world. The data collected during the internship will form a fundaments for future genetic studies, including GWAS and RNAseq experiments, to identify the genetic components underlying changes in growth dynamics of individual organs, as well as changes in overall plant architecture. This will provide new insight into breeding targets and future strategies to ensure food security in changing climate.
Faculty advisor: Magdalena Julkowska
The chemical ecology of plant communication - Kessler Lab, Cornell
Project description:
Our Lab studies the chemical and molecular mechanisms, ecological consequences and evolutionary aspects of plant induced responses to herbivory. As a specific focus, the group tries to understand how plant chemistry functions as a vehicle of information transfer between organisms and so affects ecological and evolutionary processes. The group’s research includes a number of basic and applied study systems in North America, Kenya, Peru, Costa Rica, and Colombia.
Locally we work on the chemical ecology of tall goldenrod, Solidago altissima, with a specific focus on plant communication and how chemical information affects population and community dynamics. More recently, we have started to understand how soil microbial communities affect plant chemistry and so the plants’ interactions with other organisms.
Moreover, the lab studies ways of applying chemical ecological mechanisms for more sustainable pest control and preservation of ecosystem functions in agriculture.
Research in the lab typically involves field and laboratory bioassays with plants, insects and microbes as well as chemical analytical methods.
Faculty Advisor: André Kessler
Social, ethical, and public engagement issues - Lewenstein Lab, Cornell
Project description:
The Social, Ethical, and Public Engagement (SEPE) component of CROPPS is an integral part of the overall project, providing a way to include social systems in the overall set of genomic, plant, soil, climate, and technological systems that shape research on digital biology. The goal of the SEPE component is to identify social and ethical issues and facilitate public engagement around those issues. Public engagement goes beyond “outreach,” and includes listening to concerns expressed by multiple stakeholders and public audiences, then feeding those concerns back into the research process. For example, as rural communities identify limited bandwidth as a concern both for the operation of digital biology and for broader social progress, researchers might design networked systems that include social support beyond their more specific needs.
Faculty advisor: Bruce Lewenstein
Seed-free plant genomics and symbioses– Fay-Wei Li Lab, BTI
Project description:
The Li lab uses genomic tools to investigate the evolution and biology of seed-free plants: ferns, fern allies, and bryophytes (mosses, liverworts, and hornworts). We are particularly interested in their symbioses with N2-fixing cyanobacteria, which provide plants with their own source of nitrogen fertilizer. We aim to understand the evolution and mechanisms of plant-cyanobacteria symbioses, and the results of which will lay the foundation for future engineering of “self-fertilizing” crops with less reliance on synthetic nitrogen fertilizers. To this end, we are surveying the diversity of cyanobacterial symbionts in hornworts, and using RNA-sequencing to uncover putative symbiosis-related genes.
Faculty advisor: Fay-Wei Li
Molecular and genetic approaches for improving crop nutritional quality traits – Li Li Lab, USDA
Project description:
Micronutrient deficiency and diet-related chronic diseases are global health problems. Improving micronutrient and phytonutrient contents in our food crops can help alleviate these problems. Research programs in Li lab focuses on discovering new genes that control phytonutrient and micronutrient levels in crops, understanding how plants regulate the accumulation of those compounds, and employing plant biotechnology and genetic methods to develop nutrient enriched crops. Current research projects are mainly in the areas of carotenoids (provitamin A, macular pigment, and antioxidant compounds), flavonoids (antioxidant metabolites), and selenium (essential micronutrient). We use various crops along with Arabidopsis as model systems and employ a combination of genetic, molecular, biochemical, physiological, and omics experiments in our research. We aim to develop novel strategies and new genetic tools to enrich those health beneficial compounds in our food crops.
Faculty advisor: Li Li
Pollinator health - pesticide, pathogen, and nutritional stress on bees - McArt Lab, Cornell
Project description:
Why are pollinator populations declining and what can we do about it? These are core research motivations in the McArt lab. Some current projects include: 1) Understanding pathogen transmission in plant-pollinator networks. We’ve recently found that ~20% of individual flowers have bee pathogens on them (!) and are working to understand how disease spreads in bee communities via bee-flower visitation networks. 2) Investigating how fungicides impact bees during pollination of apple. We’re unraveling a complex system where interactions between fungicides, insecticides, bee microbiota, and pathogens are all at play. 3) Understanding how pollinator populations respond to mass flowering events in agricultural systems and habitat enhancements (e.g., large wildflower plantings underneath solar panels at new solar power facilities).
Approaches in our lab typically involve field and/or lab research with bees, chemical analyses (HPLC, etc.), and molecular techniques (PCR, etc.).
Data-driven approaches to unravel and engineer plant biochemistry - Moghe Lab, Cornell
Project Description:
The Moghe Lab is fascinated by the diversity in plant metabolites and the genetic and evolutionary mechanisms that create it. Given the rapid pace of new data generation in today’s big data world, we seek to use a combination of bioinformatic and biochemical approaches to unravel plant metabolism, in species such as tomato, sweet potato and Brachypodium distachyon. Students interning in the lab will primarily code in Python and/or R, and will learn advanced genomic, phylogenetic, machine learning and protein structural analysis algorithms. The computational component is supported robustly by experimentation, where we use approaches such as enzyme assays, mutagenesis, and ultrahigh resolution mass spectrometry. Previous summer students in the lab have successfully been co-authors on publications, speaking to the high calibre of students in the REU program and those entering our lab!
Faculty Advisor: Gaurav Moghe
Bioinformatics and genomics - Mueller Lab, BTI
Project Description:
Interns in the Mueller lab work on a variety of bioinformatics and genomics projects and gain experience in the following areas: genome assembly, structural and functional annotation, biochemical pathways, comparative genomics, ontology development and data presentation and visualization.
Faculty Advisor: Lukas Mueller
Defense mechanisms in maize, with a focus on mycotoxigenic fungi - Nelson Lab, Cornell
Integrated field, greenhouse and lab studies to reduce the vulnerability of maize to fungi that cause disease
Project description:
Like other crops, maize is attacked by diverse microbes, including micro-fungi that produce toxic compounds. These toxins, including aflatoxin, fumonisin and deoxynivalenol, contaminate staple crops around the world and pose health threats to vulnerable human and animal populations. The Nelson lab is working to understand the genetic architecture of disease resistance in maize and sorghum as well as the mechanisms that enhance or reduce toxin accumulation in crops before and after harvest. We also contribute to plant disease management efforts in international contexts. We work at Cornell, with collaborating labs in North Carolina and Mississippi, and with collaborating teams in India, Tanzania and Kenya.
Faculty advisor: Rebecca Nelson
Dissecting the identity and evolution of meiotic recombination hotspots using genomics and computational biology - Pawlowski Lab, Cornell
Project description:
The goal of the Pawlowski lab is to understand the basis of inheritance in plants by studying the mechanisms governing recombination in meiosis. Meiosis is a specialized type of cell division that leads to the production of gametes. During meiosis, homologous chromosomes, one from the mother and the other one from the father, pair with each other and exchange parts in the process of recombination, which is essential for accurate transmission of genetic material from parents to progeny and for generating genetic variation. Our research combines computational biology as well as genomics methods to identify sites in the genome where recombination takes places and study their evolution. These basic studies provide means to investigating how meiotic processes can be modified to improve plant breeding methods by targeting recombination to desired sites in the genome. .
Faculty advisor: Wojtek Pawlowski
The role of membrane transport in root abiotic stress responses – Pineros Lab, USDA/Cornell
Project description:
Roots are the essential organ for plant nutrition, absorbing water and nutrients. Research in the Pineros lab focuses on the role of two distinct, but complementary aspects of root biology and plant adaptation to environmental stresses: root system architecture and membrane transport. Our goal is to understand the physiological and molecular processes underlying plant abiotic stress responses, as well as mineral nutrition-related processes. Current projects focus on A) structural and functional studies on membrane transporters that underlie Al resistance responses in crops by mediating Al exclusion or internal detoxification, and B) determining the mechanisms underlying the expression and regulation of these membrane transporters. The outcome of this research will provide a new framework for identifying molecular determinants that confer high levels of Al resistance, with the ultimate goal of “engineering” their functional characteristics to enhance the plant’s adaptation responses.
Faculty advisor: Miguel Pineros
Landscape simplification affects plant traits by mediating shifts in the insect community - Poveda Lab, Cornell
Project description:
Our research group studies the ecology of plant-insect interactions in agricultural systems and their interface with natural systems. We focus on two main themes: 1) The effect of diversity at local and landscape scales on ecosystem (dis)services essential for agricultural systems, including pollination, herbivory, biological control, and ultimately yield, and 2) the ecological, physiological, and genetic mechanisms of plant tolerance and resistance traits in agricultural crops. This summer (2022), we are focusing on a project where we are testing how agricultural land-use change alters insect communities and if this results in trait adaptation in local plant populations. Preliminary bioassay data suggest that plants from agriculturally dominant landscapes are more palatable to herbivorous insects and have greater inducibility than plants sourced from landscapes with more natural land cover. Students collaborating on this project will assist with chemical analyses to connect the bioassay results with the plant chemistry to identify the mechanisms driving these patterns.
Faculty advisor: Katja Poveda
Chemical Ecology, Plant-Pollinator Interactions and Multi-Modal Behavior - Raguso Lab, Cornell
Project description:
My students and I study the full spectrum of chemically mediated interactions between flowering plants and their insect pollinators, including typical nectar- or pollen-based systems, obligate mutualism, mimicry and deception. These studies have compelled us to develop broader interests in the evolution of signals and communication, exploring the links between chemical signals and nutrition, physiology and foraging decisions. Our study of chemically-mediated interactions has expanded to include the impact of third parties (microbial symbionts, parasites and predators), and to apply what we have learned in pollination systems to those of host specificity and biological control, including recent work on yeasts, bacteria and the spotted wing drosophila fly.
Our laboratory environment provides students with opportunities to design and perform behavioral bioassays, to learn analytical chemistry, electrophysiology, dietary manipulation and performance assays with several species of insects, plants and microbes. Learn more about our projects, publications and lab history at: https://ragusolab.weebly.com/
Faculty advisor: Robert Raguso
Nuclear architecture and epigenetic regulation of the genome - Richards Lab, BTI
Project Description:
The Richards lab studies epigenetics – the information content of the genome layered on top of the primary nucleotide sequence. The most intensely studied epigenetic codes are those embedded in the DNA, such as cytosine methylation, or involving post-translational modification of the histone proteins that comprise the nucleosomes. A less-studied aspect of epigenetics is the three-dimensional organization of the genome and the effect of alternate spatial configurations on gene expression. Our studies are focused on this higher level of epigenetic regulation with an emphasis on how nuclear structure impacts, and is shaped by, this regulation. In collaboration with the Lammerding lab at Cornell, we have support from the National Science Foundation to examine how genome size and epigenetic codes, such as cytosine methylation and histone modification, alter nuclear biomechanics. These studies are being carried out using insect cells, as well as plant genetic models
Faculty Advisor: Eric Richards
Cell size and sepal size in Arabidopsis - Roeder Lab, Cornell
Project Description:
Size is a critical property of plants, yet we know little about how it is controlled. In the Roeder laboratory, we ask how does a cell know how big to be and how does the whole organ (e.g. leaf, sepal or petal) made up of many cells know how big to be. To answer these questions we image living plants on a confocal microscope and measure the growth of cells, examine the cell division pattern, and quantify fluorescent proteins expressed.
Faculty Advisor: Adrienne Roeder
Real-time soil health and rhizosphere root phenotyping using 3D Printed Soil Swimming Robot (ROSESCOPE) - Rob Shepherd and Taryn Bauerle
Project Description
Soil, the microbiome, and plant roots represent a critical frontier in terrestrial carbon sequestration. The physics, chemistry, and biology in this zone, which play central roles in global nitrogen, carbon, and water cycles, largely drive plant productivity. The opacity of soil, coupled with the heterogeneity and dynamic nature of the soil-microbe-root system, has severely limited in situ studies and precise interventions for soil and plant management. In this project, we are developing a 3D printed soft robot that that navigates in soils with electronic and optical sensors and fluidic paths to deliver and extract water-soluble chemicals. The robotic design principles are based on an auger head for breaking the soil, multimodule actuators that lead the navigation, and a tendon drive system for steering and anchorage. A student who will be jointly supervised by Rob Shepherd and Taryn Bauerle will work on testing and experimentation of ROSESCOPE in collaboration with postdocs and graduate students. The specific tasks will involve sensor integration and biophysical experimentation in real-time with the robot. The student working on these experiments would learn to use advanced robotic tools such as 3D printing, circuit design, and basic robotic control in Shepherd’s lab, as well as developing a basic understanding of plant physiology and key parameters of plant and soil health while conducting greenhouse and larger-scale agricultural field experiments in Bauerle’s lab.
Faculty advisors:
Evolution of floral traits in a California native plant lineage (Calochortus) - Specht Lab, Cornell
Project description:
Calochortus (Liliaceae) is a large genus (75 spp.) of bulbous herbs with flowers of kaleidoscopic variety and exquisite complexity. It has a center of diversity in California and ranges north to British Columbia, west to the Dakotas, and south to Mexico and Guatemala. Calochortus has undergone striking radiations in flower morphology, habitat, and substrate preference, and most taxa have narrow ranges. Floral syndromes include Mariposas with large, brightly colored, tulip-like blossoms with an erect perianth (tepals); Cat’s Ears with a smaller, spreading perianth densely covered with trichomes; Star Tulips with spreading, mostly glabrous tepals; and Fairy Lanterns with closed, globular nodding flowers. Calochortus petals are marked by unique, often brightly colored glands. Species inhabit deserts, grasslands, chaparral, meadows, vernal pools, springs, montane woodlands, and forest understories. One quarter of all species occur on or are limited to serpentine; nearly as many are federally endangered or extinct. Several species are visited by a wide range of pollinators, while others attract a narrow range of visitors.
Given the diversity of habitats, geographic ranges, and floral forms seen in Calochortus, a well-resolved and densely sampled phylogeny would provide the opportunity to address many questions at the interface of ecology, evolution, and biogeography: Are species with similar floral syndromes each other’s closest relatives, or have such syndromes arisen multiple times independently? What is the adaptive significance and developmental origin of each floral syndrome? Has the ability to tolerate serpentine evolved more than once within and among clades? What has been the historical pattern of geographic spread within the genus? Do closely related species occupy similar ecological distributions?
The selected student will work with graduate student Adriana Hernandez to develop a phylogeny for Calochortus and to investigate gene flow and diversification in floral form among various species native to California and Mexico.
Faculty Advisor: Chelsea Specht
Chloroplast biology - Stern Lab, BTI
Project Description:
The Stern laboratory focuses on photosynthetic carbon assimilation and gene expression mechanisms in the chloroplast, where photosynthesis takes place. We use molecular genetic techniques to test hypotheses for increasing plant performance and photosynthetic efficiency by creating and analyzing transgenic plants. Our gene expression work focuses on. A key ribonuclease, RNase J, which exerts quality control over the transcript population of the chloroplast, and is itself essential for plant embryo development and viability.
Faculty Advisor: David Stern
Real-time monitoring of aphid feeding using AquaDust - Abe Stroock (Chemical and Biomolecular Engineering) and Georg Jander (Boyce Thompson Institute)
Project Description
Aphids feed on plants by inserting their slender mouthparts into individual phloem sieve elements. Over time, the removal of phloem sap by aphids causes water stress, which can be observed as loss of leaf turgor. A novel hydrogel nanoreporter (AquaDust; Jain et al, 2021, https://doi.org/10.1073/pnas.2008276118), which can be infiltrated into the apoplastic space of leaves, makes it possible to observe spatially resolved changes in leaf water relations at a microscopic scale. A student who will be jointly supervised by Abe Stroock and Georg Jander will use AquaDust to investigate the time course of aphid-induced changes in leaf water potential. The expected results of these experiments may provide a visible indicator of aphid infestations in agricultural settings, an assay to investigate natural variation in plant resistance to aphid feeding, and an basis to study the elusive biophysical mechanisms of phloem loading in the leaf.
Faculty advisors:
Plant-Insect Ecology - Thaler Lab, Cornell
Project Description:
Dr. Thaler’s lab goals are to develop a predictive framework for understanding the complex interactions that occur between plant and insect species. Studies of fundamental ecological processes, in both agricultural and wild systems, can provide insight into controlling insect pests and understanding the natural world. Thaler’s research focuses on ecological interactions between plants, herbivores, and carnivores in agricultural and wild Solanaceous plants. Current research projects focus on understanding the non-consumptive effects of predators on prey; how plants balance interactions between mutualists and antagonists such as pollinators and herbivores, and understanding how plants integrate their defenses against multiple attackers.
Faculty Advisor:
Jennifer Thaler
Biotechnological approaches to accelerate improvement of underutilized plant species - Van Eck Lab, BTI
Project Description:
Research in the Van Eck lab is focused on development of genetic engineering and gene editing approaches to support crop improvement efforts. A current focus of her work is investigation of strategies to accelerate improvement of underutilized plant species and orphan crops to diversify our food supply. By applying genetic engineering and gene editing of groundcherry and goldenberry as proof-of-concept, she has demonstrated the feasibility of targeting key genes for domestication traits to tame the wild nature of a plant species and increase its likelihood of adoption into large-scale agricultural production.
Faculty Advisor: Joyce Van Eck
Protein degradation in chloroplasts; determinants of the life-time of chloroplast proteins - Van Wijk Lab, Cornell
Project Description:
Complexity increases as you follow along the “central dogma” of biology. Model plants, such as Arabidopsis thaliana, have ~27,000 protein-coding genes (DNA) which encode several-fold more transcripts (RNA), which in turn encode hundreds of thousands of proteins. Further, proteins can be chemically modified to yield even greater complexity. Understanding how plants, or any organism, can manage such daunting complexity at the protein level requires the study of protein synthesis, maintenance, modification, and removal; processes that collectively constitute “proteostasis”. The van Wijk lab primarily focuses on the process of (selective) protein removal, called proteolysis. We focus on proteolysis in the plastid; an organelle found in al photosynthetic eukaryotes, including diatoms, algae, crops and trees but also in malaria-causing parasites (Plasmodium). Working with plastids (chloroplasts) is advantageous because of its reduced proteome complexity (~10% of the entire plant proteome) and because the mechanisms of protein degradation are distinct from that of the well-studied proteasomal degradation system in the cytosol of plants. We devote most of our resources towards studying the “natural” substrates and substrate selection mechanisms of the CLP Protease in the model plant species Arabidopsis thaliana, the most abundant protease in plastids. We employ a wide range of techniques, including molecular biology and engineering in Arabidopsis, phenotyping, protein biochemistry, mass spectrometry, recombinant technology in E. coli and bioinformatics. Broadly, we expect that an improved understanding of plastid proteolysis could lead to meaningful technologies with applications in agriculture and medicine.
Faculty Advisor: Klaas Van Wijk
Long distance micronutrient signaling and their role in reproduction and seed nutritional quality in plants– Vatamaniuk Lab, Cornell.
Project Description:
The global demand for high-yield grain crops is increasing due to the current trend of population growth, global climate change, and environmental pollution. In this regard, micronutrients such as iron and copper are required for the growth and development of all organisms including plants and humans. These elements, however, are toxic when are accumulated in cells in access. Thus plants tightly regulate copper and iron uptake from the soil to avoid deficiency while precluding toxicity. This regulation involves the transcriptional control of genes mediating copper and iron uptake from the soil, root-to-shoot partitioning and shoot-to-root signaling of copper and iron status to accommodate the demands of the growing shoot. Many of the mechanisms involved in metal transport, its regulation and signaling as well as micronutrient utilization for ensuring successful developmental programs including fertility are not well understood.
Project 1: Copper transport, it’s regulation, and influence on pollen fertility.
Using RNA-seq analysis, we identified a novel transcription factor, CITF1 (Cu-deficiency Induced Transcription Factor1), that is strongly upregulated in Arabidopsis thaliana flowers subjected to copper deficiency. We demonstrated that CITF1 regulates copper uptake into roots and delivery to flowers and is required for normal plant growth under copper deficiency. We found that CITF1 acts together with a master regulator of copper homeostasis, SPL7, and the function of both is required for copper delivery to anthers and pollen fertility. We now aim to identify the sites of copper action in anthers and pollen, the role played by SPL7 and CITF1 in pollen development, SPL7, and CITF1 transcriptional regulatory networks and transport processes governing copper homeostasis in A. thaliana. We are also analyzing SPL7 and CITF1 pathways in a globally important crop, wheat, and its proxy, a model grass species, Brachypodium distachyon.
Project 2: Shoot-to-root signaling of iron deficiency
Despite significant progress in the understanding of how plants acquire iron from the soil and how iron is mobilized within the plant, not much is known about how shoots communicate their iron status to the root. We are using A. thaliana iron deficiency signaling mutants to address the question of the nature of systemic iron deficiency signal(s), its interactions with sensors in different tissues and cell types as well as the signal propagation to root epidermal cells to trigger transcriptional iron deficiency responses.
Faculty Advisor: Olena Vatamaniuk
Internships are funded by the National Science Foundation, Research Experiences for Undergraduates Award #1358843, individual faculty grants, and the generosity of donors including the Emerson Foundation , Ithaca Garden Club, John Ben Snow, the Legacy Foundation of Tompkins County, Rheonix, Triad Foundation Inc, Yunis Realty , and many individual donors.